Unveiling the Marvels of Elastic Body Simulations
4 min read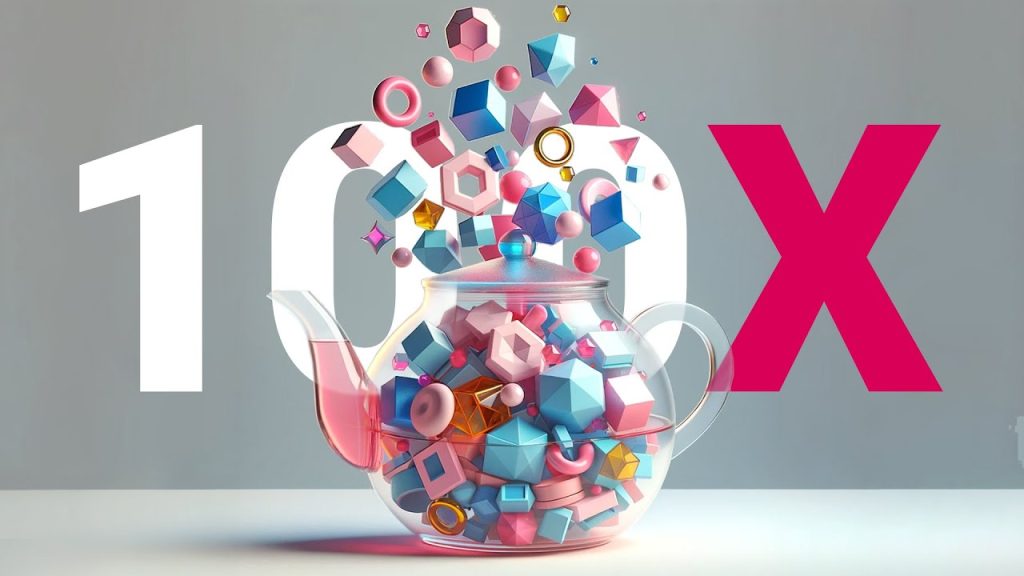
Ever thought about how squishy balls would behave inside a teapot? Imagine watching them bounce around, endlessly adding more to the mix. This simulation mirrors a crowded airport with a million people. Sounds chaotic, right?
In a mesmerizing display, these elastic interactions unfold in astonishing complexity. Overcoming the nightmare of real-life simulations, this video explores the speed and beauty of computational science. Hold on tight as we dive into a world of octopi, armadillos, and more.
Simulating Elastic Bodies
Imagine putting a bunch of elastic balls inside a teapot and watching them bounce around. Over time, more balls are added, making the simulation even more complex. The beauty of this simulation lies in how each ball interacts with another, requiring calculations for a million collisions. This kind of simulation is a marvel of science. Picture an airport crowded with a million people, all bumping into each other; that’s how chaotic it would be.
Despite the complexity, the simulation remains possible. The visual appeal of these bouncing balls is nothing short of mesmerizing. Simulating such detailed interactions would be a nightmare in real life, but here, it’s achieved seamlessly. The video promises to reveal how fast these simulations are performed, hinting at an astonishing speed.
Octopi and Armadillos Experiment
The next experiment involves even more complex interactions, featuring octopi and armadillos. Visualize the glass enclosure packed with these creatures, all pressing against each other. The challenge here is immense, as each elastic material influences the others, creating a wave-like behavior.
The difficulty of this simulation lies in modeling the bottom layer of objects accurately. You can’t just ignore the ones at the bottom because they still play a crucial role. This experiment showcases the intricate dynamics of elastic materials under pressure. The layers of interactions make this a fascinating yet challenging task.
Pushing Limits with Armadillos
One of the highlights includes flattening a virtual armadillo and watching it return to its original shape. This visual test demonstrates the simulator’s capability to handle extreme deformations while maintaining accuracy.
The experiment also includes putting the simulation through stressful conditions. The purpose is to see if the simulator remains stable. To everyone’s amazement, it does. Stability under such extreme conditions is remarkable.
In another experiment, the armadillo is pulled in various directions until it seems the simulator might fail. But it doesn’t. Instead, it remains perfectly stable, proving the simulator’s robustness. These tests show how advanced this technology has become.
Friction and Topological Changes
This simulator isn’t just about elasticity; it can also handle different friction levels and even changes in the material’s structure, like tears. This versatility is one of the most impressive aspects of the technology.
One demonstration involves a small cube and a much larger one. When subjected to pressure, older techniques would fail, but this new method succeeds. It can simulate the larger cube being pushed out of the way correctly, demonstrating its strength.
These experiments are not just for show. They highlight the simulator’s capacity to handle real-world physics scenarios. The ability to simulate complex changes, like different friction coefficients and material tears, signifies the broad applicability of this technology.
The Magic Behind the Simulation
The secret to this advanced simulation lies in breaking down a large problem into smaller, manageable ones. Techniques like Gauss-Seidel iterations help solve these smaller problems independently, akin to fixing a chair while sitting on it. This allows for efficient and accurate simulations.
This technique has a history of being used in various applications, from fluid simulations to complex structural analyses. By solving smaller parts of the problem individually, the overall simulation runs more smoothly.
The beauty of this technique is not just in its efficiency but also in its adaptability. Whether simulating fluids or elastic bodies, the approach ensures stable and accurate results. It’s a testament to how far computational simulations have come.
Speed and Performance
One of the most impressive aspects of this new technique is its speed. While older methods might take hours or days, this simulation runs in just a few seconds per frame. That’s incredibly fast for such complex calculations.
When measured against previous techniques, this new method isn’t just slightly faster; it’s exponentially quicker. It’s not just twice or three times as fast but potentially up to 1000 times faster, which is extraordinary.
This significant boost in speed doesn’t come at the cost of stability or accuracy. The technique manages to be both fast and reliable, proving its worth in advanced simulations. It’s a groundbreaking achievement in the field of computational science.
A Bright Future for Simulations
This new technique paves the way for future advancements in simulation technology. Its ability to handle complex interactions, different materials, and topological changes sets a new standard.
Researchers and scientists can now simulate real-world scenarios more accurately and efficiently than ever before. This opens up new possibilities for innovation and discovery in various fields.
In summary, this groundbreaking simulation technology not only amazes with its visual appeal but also with its efficiency and stability. The ability to simulate complex elastic interactions with such speed is revolutionary.
From virtual armadillos to intricate body simulations, the technology proves its robustness and versatility. It’s a marvel of computational science, pushing the boundaries of what’s possible.
This advancement signals a bright future for researchers and scientists, providing a powerful tool to explore real-world scenarios with unprecedented accuracy and speed.